User:Gregory Hoeprich/Sandbox 1
From Proteopedia
| |||||||||
1c1g, resolution 7.00Å () | |||||||||
---|---|---|---|---|---|---|---|---|---|
| |||||||||
| |||||||||
| |||||||||
Resources: | FirstGlance, OCA, RCSB, PDBsum | ||||||||
Coordinates: | save as pdb, mmCIF, xml |
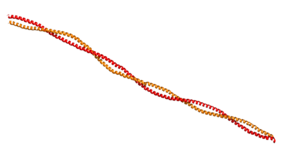
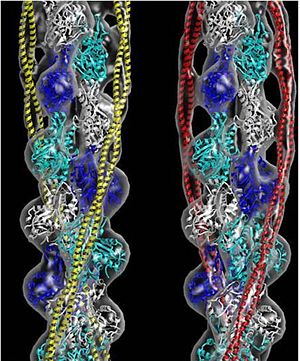
Its role in muscle mechanics has been well established as it is a major regulatory component of the contractile apparatus, but its role in non-muscle systems is becoming evermore clear. In mammals, there are at least 40 known isoforms, which are generated by alternative splicing of multiple genes[1][2]. These isoforms in non-muscle systems contribute to actin's stability by increasing filament rigidity and protecting the filament from actin severing proteins, like gelsolin or cofilin. The different isoforms also aid in recruitment of various proteins, including myosin (a family of molecular motors)[4][5]. The reason for tropomyosin's diversity is not well known, but it is thought to exist to function at different developmental stages in some species as well as function in specific cells of higher multicellular organisms[1].
The details of its structure/function relationship defines its role in different cell systems and disease states, this page further discuss tropomyosin's structure and function.
Contents |
Tropomyosin's Structure
- Class: coiled-coil
- Fold: parallel coiled-coil
- Superfamily: tropomyosin
- Family: pig [1c1g]
|
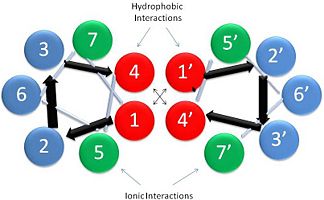
This coiled-coil domain is represented as a helical wheel diagram (see right), whereby the four amino acids (two from each alpha chain) that are adjacent to each other contribute to a , represented in red, while the four amino acids (two from each alpha chain) flanking the hydrophobic core provide an ionic interaction, or salt-bridge represented as green. The can be observed by observing chain A, residue 26, which is a glutamic acid and forms a salt bridge with an arginine, 305 on Chain B. This weak stabilizing interaction has both and electrostatic interaction, via the ions, and two hydrogen bonds between the residues. This extra stability aids in its left-handed super-coil conformation. The two hydrogen bonds between the residues have an average distance of 2.85Å. Combined the hydrophobic and ionic interactions contribute significantly to the stability of the dimer[1][3].
The amino acids from each alpha chain interact with each other uniquely in that side chains of the helices interfacing will pack together in a knobs in holes arrangement (i + i+4 ridges fit into i + i+4 grooves)[1]. This is accomplished by the ridges of one helix fitting between the grooves (between the hydrophobic amino acids in position a & d, see helical wheel diagram) of the adjacent helix. This packing further allows for the two alpha helices to wrap tightly around each other in a left handed supercoil conformation further stabilizing the tropomyosin dimer[1].
|
Post-Translational Modifications
There are two types of post-translational modifications to tropomyosin: phosphorylation acetylation[1]. Phosphorylation occurs on amino acid [1]. This phosphorylation is occurs as a result of oxidative stress, which is associated with actin remodeling and recruitment of additional tropomyosin into stress fibers[1]. The acetylation occurs on the N-terminus of the N-terminal methionine, which is essential for: coiled-coil stability, overlap formation and actin binding[2][1].
Evolutionary Conservation
Tropomyosin is highly conserved actin binding protein, which is found in Eukarya from the animal kingdom to yeast, with the exception of plants[1]. The earliest characterization of tropomyosin gene lineage was in yeast (budding and fission yeast)[1][6]. These genes are known as TPM1 and TPM2, respectively, and share 64.5% sequence identity[1][6]. As we move away from unicellular organisms and into multicellular invertebrates, tropomyosin genes in nematodes slightly diverge from yeast, but have 85-90% sequence identity between their genes[1]. Further analysis for vertebrates show there are four genes that generate over 40 known mammalian isoforms of tropomyosin, which are synthesized by exon splicing[1]. The slight evolution change of tropomyosin has occurred as a result of the increasing need of tropomyosin to function in different systems, but tropomyosin has evolutionarily stayed well conserved because of the basic structural pressures imposed on the protein[1]. It is interesting to note, the region with the least conservation has been in the N and C terminus. This is the result of head-tail interactions changing to accommodate different polymer confirmations along actin for different functions[1]. (To see this evolutionary conservation, go to the top right image of the web page and click on the "show" link, which is to the right of "Evolutionary Conservation".)
Tropomyosin's Function: Muscle and Non-Muscle Systems
Muscle tissue is comprised of many muscle fibers or cells. Those muscle fibers consist of myofibrils that contain a series of contractile units called sarcomeres. Within this unit contains thick filaments, comprised mainly of myosin, and thin filaments, which contain actin, tropomyosin and troponin. Tropomyosin in striated muscle systems (skeletal and cardiac) acts to inhibit the myosin cross-bridges from binding to the myosin binding site on thin filaments, this tropomyosin state is in the "Blocked" position[7]. When the muscle is stimulated, there is a rise in intracellular calcium stemming from a cascade of cellular processes. As the calcium is bathing the sarcomere, it will bind to the troponin complex, which is bound to both actin and tropomyosin[8]. The troponin will displace the tropomyosin from a "Blocked" to a "Closed" position[7]. This transition allows the myosin head to interact weakly with the myosin binding site[8]. The tropomyosin is displaced to its final position, "Open" state, along actin filament as myosin binds to its site[7]. These three tropomyosin states along the filament is referred to as the three state model[7]. As the intracellular calcium concentration falls, the troponin no longer is able to displace tropomyosin and it will transition back to the "Blocked" state[7].
In non-muscle systems, as mentioned above, tropomyosin performs a wide variety of functions inside of cells. One of the specific functions tropomyosin performs within cells is recruitment of different myosin motors. Clayton et al, 2010 showed actin decorated with tropomyosin increased myosin-V (cargo transporter) motility as opposed to bare actin[4]. It was also shown that myosin-I (cargo transporter and tension senor) lost its motility function on actin decorated with tropomyosin[4]. Further evidence from a related paper, Stark et al 2010, shows tropomyosin increases the affinity of myosin-II to actin filaments[5]. So actin decorated with tropomyosin appears to spatially regulate motor activity and thus cellular function.
Solved Tropomyosin Structures
3mtu, 3mud – cTPM alpha-1 – chicken
1ic2 - cTPM alpha-1 (mutant)
2w49, 2w4u – cTnnC+cTnnT+cTnnI+cTPM alpha-1+cActin
2z5h – yTPM alpha-1 N-terminal+C-terminal+GNC4 leucine zipper+TnnT – yeast
2z5i - yTPM alpha-1 N-terminal+C-terminal+GNC4 leucine zipper
2efr, 2efs, 2d3e - rTPM alpha-1 C-terminal+GNC4 leucine zipper – rabbit
1kql - TPM alpha-1 C-terminal+GNC4 leucine zipper - rat
1mv4 - TPM alpha-1 C-terminal – rat
2g9j - TPM alpha-1 TM9A+GNC4 – rat
2b9c – TPM mid region – rat
1c1g – TPM – pig
2tma – TPM - model
References
- ↑ 1.00 1.01 1.02 1.03 1.04 1.05 1.06 1.07 1.08 1.09 1.10 1.11 1.12 1.13 1.14 1.15 1.16 1.17 1.18 1.19 1.20 1.21 1.22 1.23 1.24 Tropomyosins. I. Gunning, Peter, 1950- II. Series.[DNLM: 1. Tropomyosin. W1 AD559 v.644 2008 / WE 500 T856 2008]
- ↑ 2.0 2.1 2.2 2.3 2.4 2.5 Frye J, Klenchin VA, Rayment I. Structure of the tropomyosin overlap complex from chicken smooth muscle: insight into the diversity of N-terminal recognition . Biochemistry. 2010 Jun 15;49(23):4908-20. PMID:20465283 doi:10.1021/bi100349a
- ↑ 3.0 3.1 3.2 Whitby FG, Phillips GN Jr. Crystal structure of tropomyosin at 7 Angstroms resolution. Proteins. 2000 Jan 1;38(1):49-59. PMID:10651038
- ↑ 4.0 4.1 4.2 Clayton JE, Sammons MR, Stark BC, Hodges AR, Lord M. Differential regulation of unconventional fission yeast myosins via the actin track. Curr Biol. 2010 Aug 24;20(16):1423-31. Epub 2010 Aug 12. PMID:20705471 doi:10.1016/j.cub.2010.07.026
- ↑ 5.0 5.1 Stark BC, Sladewski TE, Pollard LW, Lord M. Tropomyosin and myosin-II cellular levels promote actomyosin ring assembly in fission yeast. Mol Biol Cell. 2010 Mar 15;21(6):989-1000. Epub 2010 Jan 28. PMID:20110347 doi:10.1091/mbc.E09-10-0852
- ↑ 6.0 6.1 Drees B, Brown C, Barrell BG, Bretscher A. Tropomyosin is essential in yeast, yet the TPM1 and TPM2 products perform distinct functions. J Cell Biol. 1995 Feb;128(3):383-92. PMID:7844152
- ↑ 7.0 7.1 7.2 7.3 7.4 Lehman W, Galinska-Rakoczy A, Hatch V, Tobacman LS, Craig R. Structural basis for the activation of muscle contraction by troponin and tropomyosin. J Mol Biol. 2009 May 15;388(4):673-81. Epub 2009 Mar 31. PMID:19341744 doi:10.1016/j.jmb.2009.03.060
- ↑ 8.0 8.1 Tyska MJ, Warshaw DM. The myosin power stroke. Cell Motil Cytoskeleton. 2002 Jan;51(1):1-15. PMID:11810692 doi:10.1002/cm.10014